Published April 2025
Ethylene oxide (EtO) is a highly reactive, explosive, colorless, and odorless gas commonly used in manufacturing of sterile medical equipment and by other industries. For example, in the United States, EtO is currently used to sterilize 50% of all medical devices. We published a comprehensive description of available EtO measurement technologies titled Emerging Technologies for Measuring Ambient Ethylene Oxide in 2022. Since then, measurement techniques have advanced.
Using spectroscopic techniques, minimum detection limits are now achievable below 0.5 parts per billion (ppb) at 1-sec time resolution, and as low as single-digit parts per trillion (ppt) with preconcentration and 15-min time resolution (1 ppt is one-thousandth of a ppb). More than ever before, selection of the most appropriate instrument(s) for measuring EtO depends on the intended use case.
In this post, we will discuss three different use-case scenarios: (1) community monitoring, (2) worker safety and exposure monitoring, and (3) industrial process or emissions monitoring.
Ambient Air Monitoring in Communities
General scientific understanding of ambient EtO background concentrations is an active area of research. In recent years, the ability to measure into the sub-100 ppt levels (or sub-0.1 ppb) has permitted observations of more pervasive background EtO levels than previously thought. A key driver of regulations enacted in 2024 and 2025 was the goal of reducing EtO exposures for industrial workers and nearby communities to levels below those corresponding to an estimated lifetime cancer risk of 100 in 1 million. As such, ambient EtO concentrations must be measured at very low levels—i.e., 1-10 ppt. This level of sensitivity is essential for evaluating estimates of community EtO exposures in the context of background concentrations, which are currently understood to vary seasonally; seasonal averages may be as low as 30 ppt or below.
The current regulatory environment for industrial facilities—discussed in a companion post by my colleague, Dana Coe, titled Recent Ethylene Oxide Regulations—underscores the growing need for ultra-sensitive instruments that can differentiate between ambient background concentrations of EtO and industrial emissions.
Worker Safety and Occupational Exposure
Real-time EtO monitoring in occupational environments usually have detection limits on the order of 100 ppb (i.e. 100,000 ppt). These instruments provide real-time information in facility process areas where EtO is regularly used. Meanwhile, many highly sensitive instruments are not certified as ‘intrinsically safe’ for use in an environment where explosive gases could be present. In such environments, instrumentation is limited to photoionization detectors and electrochemical sensors, which usually measure between 100 ppb – 1 ppm (i.e. 100,000 – 1,000,000 ppt) and can have cross-sensitivities to other gases or be influenced by environmental factors.
EPA’s January 2025 Interim Decision has tightened worker exposure limits, which will require refined monitoring and compliance approaches. Key changes to the limits and the phased compliance schedule were summarized in the Interim Decision, as shown in Table 1.
Table 1. Summary of Worker Exposure Limit, Short-Term Exposure Limit, and Action Level for EtO commercial Sterilization Facilities (as reproduced from Table 4 of Interim Registration Review Decision for EtO, page 83; with units of measure converted from ppm to ppb).
HTMLProcess<table style="width:100%">
<tr>
<th>Description</th>
<th>Current OSHA Standard</th>
<th>New EPA Limit</th>
<th>Compliance Timeline</th>
</tr>
<tr>
<td rowspan=3>Exposure Limit (8-hr time-weighted average)</td>
<td rowspan=3>1,000 ppb</td>
<td>500 ppb</td>
<td>3 years</td>
</tr>
<tr>
<td>250 ppb</td>
<td>5 years</td>
</tr>
<tr>
<td>100 ppb</td>
<td>10 years</td>
</tr>
<tr>
<td>Short-Term Exposure Limit</td>
<td>5,000 ppb (15-min time-weighted average)</td>
<td>5,000 ppb (10-min time-weighted average)</td>
<td>10 years</td>
</tr>
<tr>
<td>Action Level* (8-hr time-weighted average)</td>
<td>500 ppb</td>
<td>100 ppb</td>
<td>1 year</td>
</tr>
</table>
HTMLProcess<style>
th {
color: white;
font-weight: bold;
background-color: #1f3b57;
border: 1px solid black;
padding-top: 5px;
padding-bottom: 5px;
text-align: center;
}
table {
border-collapse: collapse;
border-spacing: 0;
}
td {
border: 1px solid black;
padding-top: 5px;
padding-bottom: 5px;
padding-left: 5px;
}
</style>
*An ‘action level’ is a concentration threshold that triggers certain compliance requirements, such as undertaking medical surveillance, providing employee training, and others.
Process Emission Measurements and Control Efficiency
For facilities that use more than 100 lbs. of EtO per year, EPA’s 2024 National Emission Standards for Hazardous Air Pollutants (NESHAP) regulation requires the use of continuous emissions monitoring systems (CEMS) to demonstrate compliance with the regulation. For this purpose, CEMS must reliably and accurately achieve 90% data capture at facility-specific, process-specific concentration ranges.
Current Measurement Capabilities
As I mentioned earlier, my colleague previously posted a discussion of available technologies for measuring EtO. Technologies have improved over the past three years, and instruments can now achieve lower detection limits (DL) and better accuracy at higher time resolutions. Current state-of-the-art measurement capabilities are summarized in the table below.
HTMLProcess<table style="width:100%">
<tr>
<th>Technique</th>
<th>Time Resolution</th>
<th>1-sec LOD</th>
<th>Best-Reported DL</th>
<th>Time for Best-Reported DL</th>
<th>Description</th>
<th>Advantages</th>
<th>Limitations</th>
</tr>
<tr>
<td>Time-of-Flight Proton Transfer Reaction Mass Spectrometry (TOF-PTR-MS)</td>
<td>Real-time</td>
<td>270-850 ppt</td>
<td>29-90 ppt</td>
<td>60 sec</td>
<td>Soft chemical ionization and high-resolution mass spectrometry for real-time EtO measurement.</td>
<td>High resolution, real-time detection, minimal fragmentation.</td>
<td>High cost, requires skilled operation, potential interferences.</td>
</tr>
<tr>
<td>Selected Ion Flow-Tube Mass Spectrometry (SIFT-MS)</td>
<td>Real-time</td>
<td>< 1,000 ppt</td>
<td>75 ppt</td>
<td>30 min</td>
<td>Chemical ionization technique allowing real-time analysis without chromatography.</td>
<td>High sensitivity, real-time quantification.</td>
<td>Potential interferences, complex operation, high cost.</td>
</tr>
<tr>
<td>Cavity Ring-Down Spectroscopy (CRDS)</td>
<td>Real-time</td>
<td>400 ppt (2 seconds)</td>
<td>100 ppt</td>
<td>300 sec, 3σ</td>
<td>Laser-based absorption technique measuring light decay time to determine EtO concentration.</td>
<td>High precision, stable over long-term use.</td>
<td>Sensitive to environmental conditions.</td>
</tr>
<tr>
<td>Preconcentration-Enhanced CRDS</td>
<td>Real-time/ 15-min analysis mode</td>
<td>1,000 ppt (5 seconds)</td>
<td>2 ppt</td>
<td>15 min</td>
<td>CRDS combined with thermal desorption to enhance sensitivity.</td>
<td>Ultra-low detection limits (~2 ppt), high precision. 11 ppt verified by EPA.</td>
<td>Requires longer sampling times (~15 min), complex operation.</td>
</tr>
<tr>
<td>Fourier-Transform Infrared Spectroscopy (FTIR)</td>
<td>Real-time</td>
<td>1,000 ppt</td>
<td>600 ppt</td>
<td>12 min</td>
<td>Infrared absorption to detect multiple compounds simultaneously, including EtO.</td>
<td>Real-time analysis, simultaneous multi-gas detection.</td>
<td>Potential interferences, requires background correction.</td>
</tr>
<tr>
<td>Mid-Infrared (IR) Spectroscopy</td>
<td>Real-time</td>
<td>1,000 -2,000 ppt</td>
<td>--</td>
<td>--</td>
<td>High-resolution tunable laser absorption in mid-IR range.</td>
<td>Ultra-portable, suitable for mobile and handheld deployments, competitive pricing.</td>
<td>Moderate detection limits compared to other methods.</td>
</tr>
<tr>
<td>Tunable Infrared Laser Direct Absorption Spectroscopy (TILDAS-FD-EtO)</td>
<td>Real-time</td>
<td>75 ppt</td>
<td>20 ppt</td>
<td>100 sec, 1σ</td>
<td>Mid-IR, tunable IR laser direct absorption spectroscopy using a multi-pass cell.</td>
<td>High sensitivity, real-time monitoring, mobile capability, can achieve single-digit detection limits at long averaging times with frequent auto backgrounds (2.3 ppt at 1.6 hours).</td>
<td>Requires frequent calibration, sensitive to environmental conditions.</td>
</tr>
<tr>
<td>Photoacoustic IR Spectroscopy</td>
<td>Real-time</td>
<td>80 ppb (i.e., 80,000 ppt)</td>
<td>--</td>
<td>--</td>
<td>Detects acoustic waves generated by EtO absorbing IR light.</td>
<td>Compact design, portable.</td>
<td>Interference from other gases and water vapor.</td>
</tr>
<tr>
<td>Photoionzation detectors</td>
<td>Real-time</td>
<td>100 - 1,000 ppb (i.e., 100,000 -1,000,000 ppt)</td>
<td>--</td>
<td>--</td>
<td>Uses high-energy ultraviolet light to ionize EtO, generating an electrical current proportional to its concentration.</td>
<td>Fast response, real-time measurements, and cost effective.</td>
<td>Non-selective (responds to other volatile organic compounds (VOCs) with similar ionization potentials), humidity interference.</td>
</tr>
<tr>
<td>Electrochemical sensors</td>
<td>Real-time/ 30-60 sec</td>
<td>100 - 1,000 ppb (i.e., 100,000 -1,000,000 ppt)</td>
<td>--</td>
<td>--</td>
<td>EtO undergoes a redox reaction at an electrode, generating a measurable electrical signal proportional to its concentration.</td>
<td>More selective than PIDs, lower power consumption, compact and cost-effective.</td>
<td>Slower response, cross-sensitivity to other gases (e.g., ozone, NO₂).</td>
</tr>
<tr>
<td>TO-15/TO-15A Canister Analysis (GC/MS)</td>
<td>Offline analysis</td>
<td>n/a</td>
<td>15 - 30 ppt</td>
<td>--</td>
<td>Air samples collected in canisters and analyzed via gas chromatography-mass spectrometry (GC-MS).</td>
<td>EPA-established, high sensitivity.</td>
<td>Requires laboratory analysis, potential biases from canister materials.</td>
</tr>
<tr>
<td>Modified OSHA Method 1010 (Sorbent Tubes + GC/MS Analysis)</td>
<td>Offline analysis</td>
<td>n/a</td>
<td>1,500 ppt</td>
<td>--</td>
<td>Uses sorbent tubes or cartridges for sampling, followed by lab-based analysis.</td>
<td>Regulatory time weighted average (TWA) method.</td>
<td>Variability in extraction efficiency, coelution with other VOCs.</td>
</tr>
</table>
HTMLProcess<style>
th {
color: white;
font-weight: bold;
background-color: #1f3b57;
border: 1px solid black;
padding-top: 5px;
padding-bottom: 5px;
text-align: center;
}
table {
border-collapse: collapse;
border-spacing: 0;
}
td {
border: 1px solid black;
padding-top: 5px;
padding-bottom: 5px;
padding-left: 5px;
}
</style>
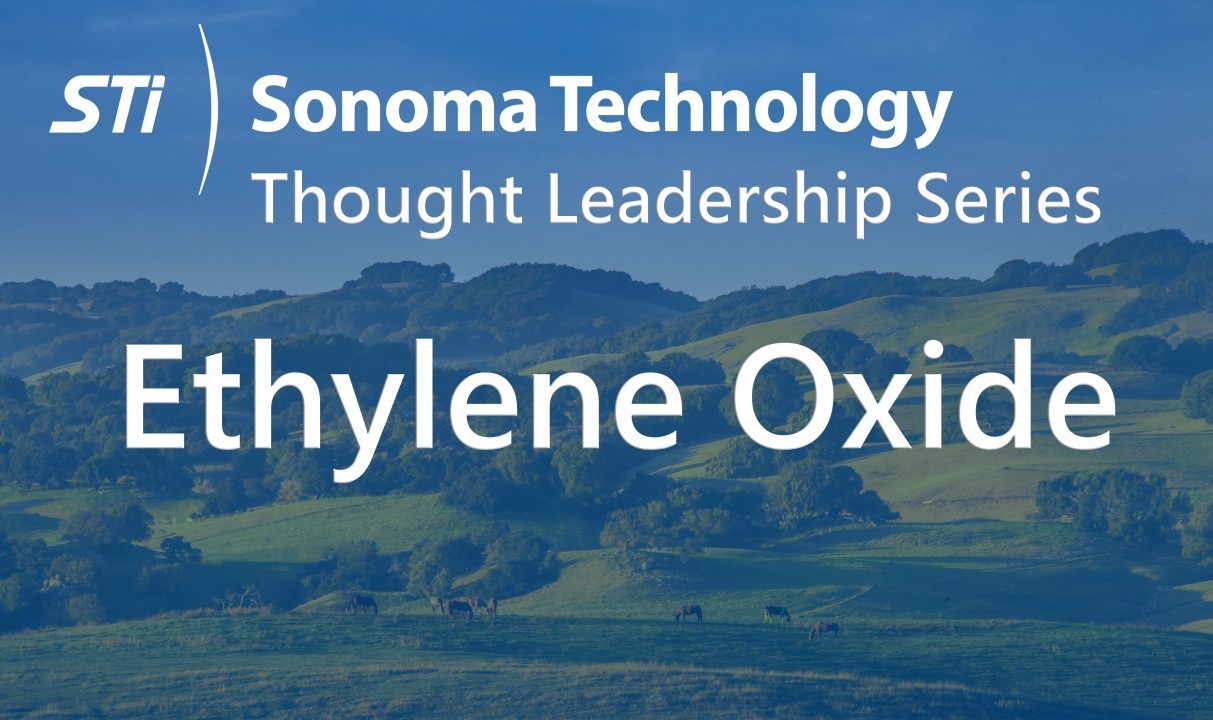
Ethan W. Emerson
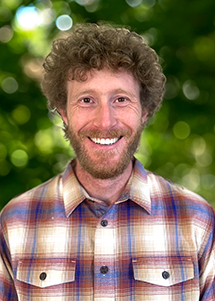